15.5: 核糖体和蛋白质合成
培养技能
- 描述蛋白质合成的不同步骤
- 讨论核糖体在蛋白质合成中的作用
蛋白质的合成比任何其他代谢过程消耗更多的细胞能量。 反过来,蛋白质比活生物体的任何其他成分(水除外)所占的质量都要大,而蛋白质几乎发挥了细胞的所有功能。 翻译或蛋白质合成过程涉及将 mRNA 信息解码为多肽产物。 氨基酸通过相互连接的肽键共价串在一起,长度从大约50个氨基酸残基到超过1,000个氨基酸残基不等。 每个单独的氨基酸都有一个氨基基(NH 2)和一个羧基(COOH)基。 当一种氨基酸的氨基与另一种氨基酸的羧基形成酰胺(即肽)键时,就会形成多肽(图15.5.1)。 这种反应由核糖体催化并产生一个水分子。
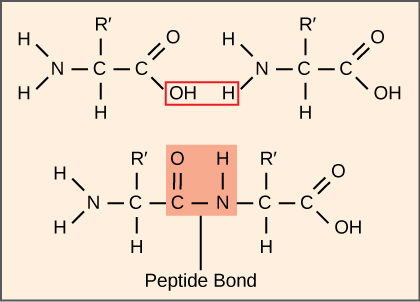
蛋白质合成机械
除了 mRNA 模板外,许多分子和大分子也参与了翻译过程。 每种成分的组成可能因物种而异;例如,核糖体可能由不同数量的 rRNA 和多肽组成,具体取决于生物体。 但是,从细菌到人体细胞,蛋白质合成机制的一般结构和功能是可比的。 翻译需要输入 mRNA 模板、核糖体、tRNA 和各种酶促因子。
核糖体
即使在 mRNA 被翻译之前,细胞就必须投入能量来构建其每个核糖体。 在大肠杆菌中,在任何给定时间,每个细胞中都存在10,000至70,000个核糖体。 核糖体是一种复杂的大分子,由结构和催化的 rRNA 以及许多不同的多肽组成。 在真核生物中,核仁完全专门用于合成和组装 rRNA。
核糖体存在于原核生物的细胞质中,也存在于真核生物的细胞质和粗糙的内质网中。 线粒体和叶绿体在基质和基质中也有自己的核糖体,它们看起来更像原核生物核糖体(并且具有相似的药物敏感性),而不是细胞质中外膜之外的核糖体。 当核糖体不合成蛋白质时,它们会分解成大亚基和小亚单位,并在翻译开始时重新结合。 在大肠杆菌中,小亚基被描述为30S,大亚基为50S,总共为70S(回想一下,Svedberg 单位不是添加剂)。 哺乳动物核糖体有一个小的40S亚基和一个大的60S亚单位,总共为80S。 小亚基负责结合 mRNA 模板,而大型亚基则按顺序结合 tRNA。 每个 mRNA 分子都由许多核糖体同时翻译,所有核糖体都朝着相同的方向合成蛋白质:从 5' 读取 mRNA 到 3',然后将多肽从 N 末端合成到 C 末端。 完整的 mRNA/多核糖体结构称为多体。
trNA
tRNA 是结构化的 RNA 分子,由 RNA 聚合酶 III 从基因中转录。 根据物种的不同,细胞质中存在 40 到 60 种类型的 tRNA。 作为适配器,特异性tRNA与mRNA模板上的序列结合,并将相应的氨基酸添加到多肽链中。 因此,tRNA实际上是将RNA语言 “转化” 为蛋白质语言的分子。
在64种可能的mRNA密码子或A、U、G和C的三联体组合中,有三个指定终止蛋白质合成,61个指定在多肽链中添加氨基酸。 在这61个中,一个密码子(AUG)也对翻译的启动进行编码。 根据遗传密码,每个 tRNA 抗密码子都可以与其中一个 mRNA 密码子进行碱基配对,然后添加氨基酸或终止翻译。 例如,如果序列 CUA 出现在正确读取框中的 mRNA 模板上,它将结合表达互补序列 GAU 的 tRNA,该序列将与氨基酸亮氨酸相关联。
作为翻译的适配分子,令人惊讶的是,tRNA能够在如此小的封装中具有如此高的特异性。 考虑一下 tRNA 需要与三个因素相互作用:1) 它们必须被正确的氨酰合成酶识别(见下文);2)它们必须被核糖体识别;3)它们必须与 mRNA 中的正确序列结合。
氨酰基 tRNA 合成酶
RNA 聚合酶 III 在 tRNA 前合成的过程只能产生适配器分子的 RNA 部分。 在 tRNA 经过处理并输出到细胞质后,必须稍后添加相应的氨基酸。 通过 tRNA “充电” 过程,每个 tRNA 分子都通过一组称为氨酰基 tRNA 合成酶的酶与其正确的氨基酸相连。 20 种氨基酸中每一种至少存在一种氨基酰基 tRNA 合成酶;氨酰基 tRNA 合成酶的确切数量因物种而异。 这些酶首先结合并水解 ATP,以催化氨基酸和一磷酸腺苷(AMP)之间的高能键;焦磷酸分子在此反应中被排出。 然后将活化的氨基酸转移到 tRNA 中,然后释放 AMP。
蛋白质合成的机制
与 mRNA 合成一样,蛋白质合成可分为三个阶段:起始阶段、伸长阶段和终止阶段。 原核生物和真核生物的翻译过程类似。 在这里,我们将探讨代表性原核生物大肠杆菌的翻译是如何发生的,并具体说明原核生物和真核翻译之间的任何区别。
开始翻译
蛋白质合成始于起始复合物的形成。 在大肠杆菌中,这种复合物涉及小型 30S 核糖体、mRNA 模板、三个起始因子(iF;IF-1、IF-2 和 IF-3)和一种特殊的引发剂 tRNA,称为tRNAMetf. The initiator tRNA interacts with the start codon AUG (or rarely, GUG), links to a formylated methionine called fMet, and can also bind IF-2. Formylated methionine is inserted by fMet−tRNAMetf at the beginning of every polypeptide chain synthesized by E. coli, but it is usually clipped off after translation is complete. When an in-frame AUG is encountered during translation elongation, a non-formylated methionine is inserted by a regular Met-tRNAMet.
In E. coli mRNA, a sequence upstream of the first AUG codon, called the Shine-Dalgarno sequence (AGGAGG), interacts with the rRNA molecules that compose the ribosome. This interaction anchors the 30S ribosomal subunit at the correct location on the mRNA template. Guanosine triphosphate (GTP), which is a purine nucleotide triphosphate, acts as an energy source during translation—both at the start of elongation and during the ribosome’s translocation.
In eukaryotes, a similar initiation complex forms, comprising mRNA, the 40S small ribosomal subunit, IFs, and nucleoside triphosphates (GTP and ATP). The charged initiator tRNA, called Met-tRNAi, does not bind fMet in eukaryotes, but is distinct from other Met-tRNAs in that it can bind IFs.
Instead of depositing at the Shine-Dalgarno sequence, the eukaryotic initiation complex recognizes the 7-methylguanosine cap at the 5' end of the mRNA. A cap-binding protein (CBP) and several other IFs assist the movement of the ribosome to the 5' cap. Once at the cap, the initiation complex tracks along the mRNA in the 5' to 3' direction, searching for the AUG start codon. Many eukaryotic mRNAs are translated from the first AUG, but this is not always the case. According to Kozak’s rules, the nucleotides around the AUG indicate whether it is the correct start codon. Kozak’s rules state that the following consensus sequence must appear around the AUG of vertebrate genes: 5'-gccRccAUGG-3'. The R (for purine) indicates a site that can be either A or G, but cannot be C or U. Essentially, the closer the sequence is to this consensus, the higher the efficiency of translation.
Once the appropriate AUG is identified, the other proteins and CBP dissociate, and the 60S subunit binds to the complex of Met-tRNAi, mRNA, and the 40S subunit. This step completes the initiation of translation in eukaryotes.
Translation, Elongation, and Termination
In prokaryotes and eukaryotes, the basics of elongation are the same, so we will review elongation from the perspective of E. coli. The 50S ribosomal subunit of E. coli consists of three compartments: the A (aminoacyl) site binds incoming charged aminoacyl tRNAs. The P (peptidyl) site binds charged tRNAs carrying amino acids that have formed peptide bonds with the growing polypeptide chain but have not yet dissociated from their corresponding tRNA. The E (exit) site releases dissociated tRNAs so that they can be recharged with free amino acids. There is one exception to this assembly line of tRNAs: in E. coli, fMet−tRNAMetf is capable of entering the P site directly without first entering the A site. Similarly, the eukaryotic Met-tRNAi, with help from other proteins of the initiation complex, binds directly to the P site. In both cases, this creates an initiation complex with a free A site ready to accept the tRNA corresponding to the first codon after the AUG.
During translation elongation, the mRNA template provides specificity. As the ribosome moves along the mRNA, each mRNA codon comes into register, and specific binding with the corresponding charged tRNA anticodon is ensured. If mRNA were not present in the elongation complex, the ribosome would bind tRNAs nonspecifically.
Elongation proceeds with charged tRNAs entering the A site and then shifting to the P site followed by the E site with each single-codon “step” of the ribosome. Ribosomal steps are induced by conformational changes that advance the ribosome by three bases in the 3' direction. The energy for each step of the ribosome is donated by an elongation factor that hydrolyzes GTP. Peptide bonds form between the amino group of the amino acid attached to the A-site tRNA and the carboxyl group of the amino acid attached to the P-site tRNA. The formation of each peptide bond is catalyzed by peptidyl transferase, an RNA-based enzyme that is integrated into the 50S ribosomal subunit. The energy for each peptide bond formation is derived from GTP hydrolysis, which is catalyzed by a separate elongation factor. The amino acid bound to the P-site tRNA is also linked to the growing polypeptide chain. As the ribosome steps across the mRNA, the former P-site tRNA enters the E site, detaches from the amino acid, and is expelled (Figure 15.5.2). Amazingly, the E. coli translation apparatus takes only 0.05 seconds to add each amino acid, meaning that a 200-amino acid protein can be translated in just 10 seconds.
Art Connection
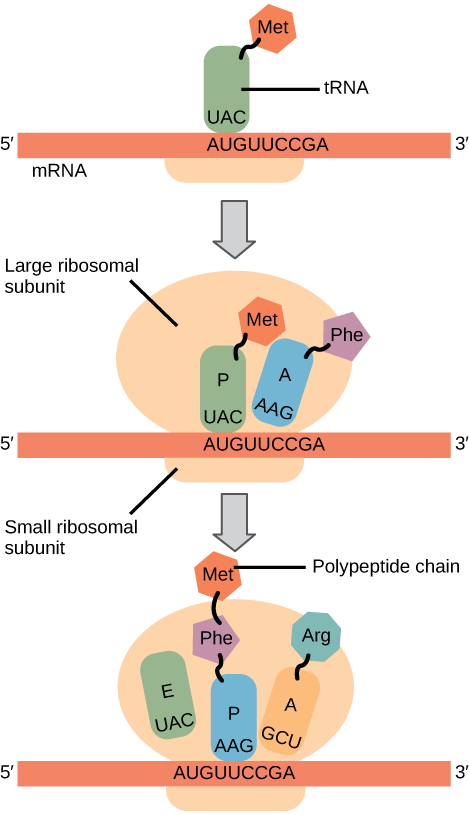
Many antibiotics inhibit bacterial protein synthesis. For example, tetracycline blocks the A site on the bacterial ribosome, and chloramphenicol blocks peptidyl transfer. What specific effect would you expect each of these antibiotics to have on protein synthesis?
Tetracycline would directly affect:
- tRNA binding to the ribosome
- ribosome assembly
- growth of the protein chain
Chloramphenicol would directly affect
- tRNA binding to the ribosome
- ribosome assembly
- growth of the protein chain
Termination of translation occurs when a nonsense codon (UAA, UAG, or UGA) is encountered. Upon aligning with the A site, these nonsense codons are recognized by release factors in prokaryotes and eukaryotes that instruct peptidyl transferase to add a water molecule to the carboxyl end of the P-site amino acid. This reaction forces the P-site amino acid to detach from its tRNA, and the newly made protein is released. The small and large ribosomal subunits dissociate from the mRNA and from each other; they are recruited almost immediately into another translation initiation complex. After many ribosomes have completed translation, the mRNA is degraded so the nucleotides can be reused in another transcription reaction.
Protein Folding, Modification, and Targeting
During and after translation, individual amino acids may be chemically modified, signal sequences may be appended, and the new protein “folds” into a distinct three-dimensional structure as a result of intramolecular interactions. A signal sequence is a short tail of amino acids that directs a protein to a specific cellular compartment. These sequences at the amino end or the carboxyl end of the protein can be thought of as the protein’s “train ticket” to its ultimate destination. Other cellular factors recognize each signal sequence and help transport the protein from the cytoplasm to its correct compartment. For instance, a specific sequence at the amino terminus will direct a protein to the mitochondria or chloroplasts (in plants). Once the protein reaches its cellular destination, the signal sequence is usually clipped off.
Many proteins fold spontaneously, but some proteins require helper molecules, called chaperones, to prevent them from aggregating during the complicated process of folding. Even if a protein is properly specified by its corresponding mRNA, it could take on a completely dysfunctional shape if abnormal temperature or pH conditions prevent it from folding correctly.
Summary
The players in translation include the mRNA template, ribosomes, tRNAs, and various enzymatic factors. The small ribosomal subunit forms on the mRNA template either at the Shine-Dalgarno sequence (prokaryotes) or the 5' cap (eukaryotes). Translation begins at the initiating AUG on the mRNA, specifying methionine. The formation of peptide bonds occurs between sequential amino acids specified by the mRNA template according to the genetic code. Charged tRNAs enter the ribosomal A site, and their amino acid bonds with the amino acid at the P site. The entire mRNA is translated in three-nucleotide “steps” of the ribosome. When a nonsense codon is encountered, a release factor binds and dissociates the components and frees the new protein. Folding of the protein occurs during and after translation.
Art Connections
Figure 15.5.2: Many antibiotics inhibit bacterial protein synthesis. For example, tetracycline blocks the A site on the bacterial ribosome, and chloramphenicol blocks peptidyl transfer. What specific effect would you expect each of these antibiotics to have on protein synthesis?
Tetracycline would directly affect:
- tRNA binding to the ribosome
- ribosome assembly
- growth of the protein chain
Chloramphenicol would directly affect
- tRNA binding to the ribosome
- ribosome assembly
- growth of the protein chain
- Answer
-
Tetracycline: a; Chloramphenicol: c.
Glossary
- aminoacyl tRNA synthetase
- enzyme that “charges” tRNA molecules by catalyzing a bond between the tRNA and a corresponding amino acid
- initiator tRNA
- in prokaryotes, called tRNAMetf; in eukaryotes, called tRNAi; a tRNA that interacts with a start codon, binds directly to the ribosome P site, and links to a special methionine to begin a polypeptide chain
- Kozak’s rules
- determines the correct initiation AUG in a eukaryotic mRNA; the following consensus sequence must appear around the AUG: 5’-GCC(purine)CCAUGG-3’; the bolded bases are most important
- peptidyl transferase
- RNA-based enzyme that is integrated into the 50S ribosomal subunit and catalyzes the formation of peptide bonds
- polysome
- mRNA molecule simultaneously being translated by many ribosomes all going in the same direction
- Shine-Dalgarno sequence
- (AGGAGG); initiates prokaryotic translation by interacting with rRNA molecules comprising the 30S ribosome
- signal sequence
- short tail of amino acids that directs a protein to a specific cellular compartment
- start codon
- AUG (or rarely, GUG) on an mRNA from which translation begins; always specifies methionine