43.6 : Fécondation et développement embryonnaire précoce
- Page ID
- 189516
Compétences à développer
- Discutez de la façon dont se
- Expliquer comment l'embryon se forme à partir du zygote
- Discuter du rôle du clivage et de la gastrulation dans le développement des animaux
Le processus par lequel un organisme passe d'un zygote unicellulaire à un organisme multicellulaire est complexe et bien régulé. Les premiers stades du développement embryonnaire sont également cruciaux pour garantir la forme physique de l'organisme.
Fertilisation
La fécondation, illustrée à la figure\(\PageIndex{1}\) a, est le processus par lequel les gamètes (un ovule et un sperme) fusionnent pour former un zygote. L'ovule et le sperme contiennent chacun un ensemble de chromosomes. Pour s'assurer que la progéniture ne possède qu'un seul ensemble diploïde complet de chromosomes, un seul spermatozoïde doit fusionner avec un ovule. Chez les mammifères, l'œuf est protégé par une couche de matrice extracellulaire composée principalement de glycoprotéines appelées zone pellucide. Lorsqu'un spermatozoïde se lie à la zone pellucide, une série d'événements biochimiques, appelés réactions acrosomales, se produisent. Chez les mammifères placentaires, l'acrosome contient des enzymes digestives qui initient la dégradation de la matrice glycoprotéique protégeant l'ovule et permettant à la membrane plasmique du sperme de fusionner avec la membrane plasmique de l'œuf, comme l'illustre la figure\(\PageIndex{1}\) b. La fusion de ces deux membranes crée une ouverture à travers dont le noyau du sperme est transféré dans l'ovule. Les membranes nucléaires de l'ovule et du sperme se décomposent et les deux génomes haploïdes se condensent pour former un génome diploïde.
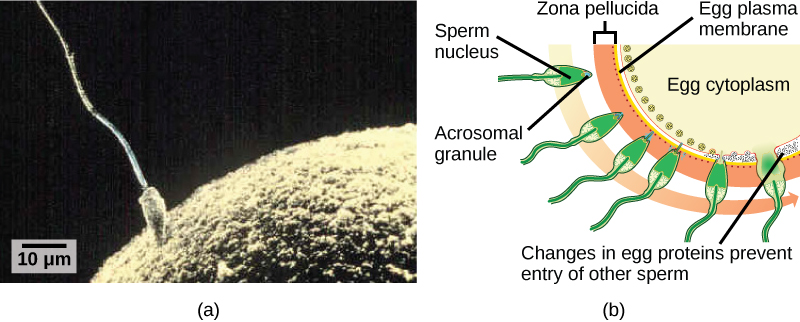
Pour s'assurer qu'un seul spermatozoïde ne féconde l'ovule, une fois que les réactions acrosomales se produisent à un endroit de la membrane de l'ovule, l'ovule libère des protéines à d'autres endroits pour empêcher les autres spermatozoïdes de fusionner avec l'ovule. Si ce mécanisme échoue, plusieurs spermatozoïdes peuvent fusionner avec l'ovule, ce qui entraîne une polyspermie. L'embryon qui en résulte n'est pas génétiquement viable et meurt en quelques jours.
Décolleté et stade Blastula
Le développement des organismes multicellulaires commence à partir d'un zygote unicellulaire, qui subit une division cellulaire rapide pour former la blastula. Les cycles multiples et rapides de division cellulaire sont appelés clivage. Le clivage est illustré dans (Figure\(\PageIndex{2}\) a). Une fois que le clivage a produit plus de 100 cellules, l'embryon est appelé blastula. La blastula est généralement une couche sphérique de cellules (le blastoderme) entourant une cavité remplie de liquide ou de jaune d'oeuf (le blastoceau). À ce stade, les mammifères forment une structure appelée blastocyste, caractérisée par une masse cellulaire interne distincte de la blastula environnante, illustrée à la Figure\(\PageIndex{2}\) b. Pendant le clivage, les cellules se divisent sans augmentation de masse ; c'est-à-dire qu'un gros zygote unicellulaire se divise en plusieurs cellules plus petites . Chaque cellule de la blastula est appelée blastomère.
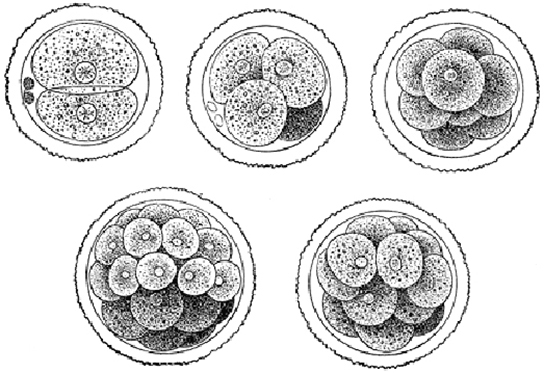
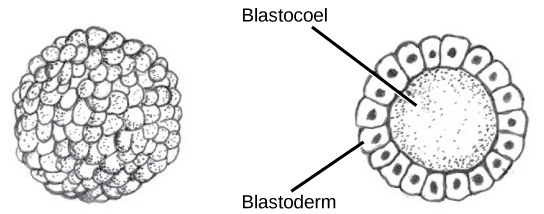
Le clivage peut avoir lieu de deux manières : clivage holoblastique (total) ou clivage méroblastique (partiel). Le type de clivage dépend de la quantité de jaune d'oeuf dans les œufs. Chez les mammifères placentaires (y compris les humains) dont la nourriture est fournie par le corps de la mère, les œufs contiennent une très petite quantité de jaune et subissent un clivage holoblastique. D'autres espèces, comme les oiseaux, dont l'œuf contient beaucoup de jaune d'oeuf pour nourrir l'embryon au cours du développement, subissent un clivage méroblastique.
Chez les mammifères, la blastula forme le blastocyste au stade suivant de développement. Ici, les cellules de la blastula se répartissent en deux couches : la masse cellulaire interne et une couche externe appelée trophoblaste. La masse cellulaire interne est également connue sous le nom d'embryoblaste et cette masse de cellules va ensuite former l'embryon. À ce stade de développement, illustré par\(\PageIndex{3}\) la figure, la masse cellulaire interne est constituée de cellules souches embryonnaires qui se différencient en différents types de cellules dont l'organisme a besoin. Le trophoblaste contribuera au placenta et nourrira l'embryon.
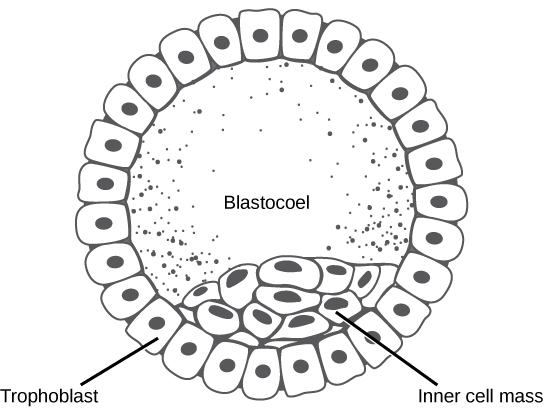
Lien vers l'apprentissage
Visitez le projet Virtual Human Embryon sur le site du Endowment for Human Development pour découvrir une page interactive qui montre les étapes du développement de l'embryon, y compris des micrographies et des images 3D rotatives.
Gastrulation
La blastula typique est une boule de cellules. La prochaine étape du développement embryonnaire est la formation du plan corporel. Les cellules de la blastula se réorganisent dans l'espace pour former trois couches de cellules. Ce processus s'appelle la gastrulation. Au cours de la gastrulation, la blastula se replie sur elle-même pour former les trois couches de cellules. Chacune de ces couches est appelée couche germinale et chaque couche germinale se différencie en différents systèmes organiques.
Les trois couches de germes, illustrées sur la figure\(\PageIndex{4}\), are the endoderm, the ectoderm, and the mesoderm. The ectoderm gives rise to the nervous system and the epidermis. The mesoderm gives rise to the muscle cells and connective tissue in the body. The endoderm gives rise to columnar cells found in the digestive system and many internal organs.
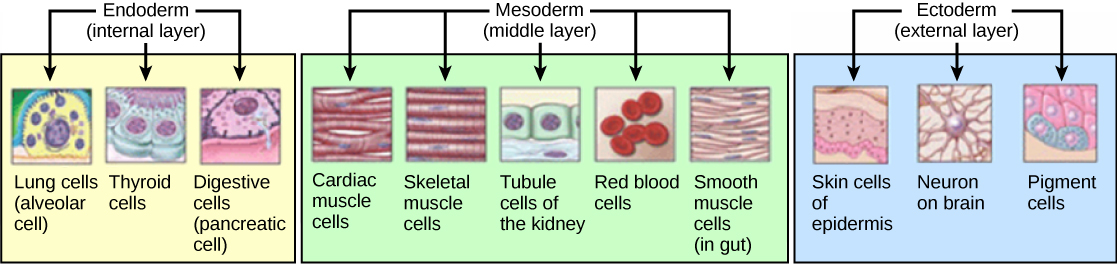
Everyday Connection: Are Designer Babies in Our Future?
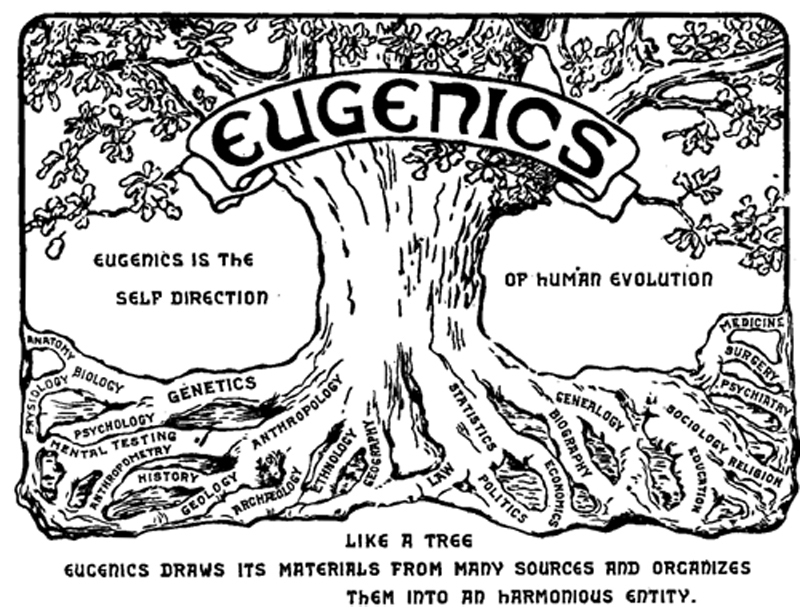
If you could prevent your child from getting a devastating genetic disease, would you do it? Would you select the sex of your child or select for their attractiveness, strength, or intelligence? How far would you go to maximize the possibility of resistance to disease? The genetic engineering of a human child, the production of "designer babies" with desirable phenotypic characteristics, was once a topic restricted to science fiction. This is the case no longer: science fiction is now overlapping into science fact. Many phenotypic choices for offspring are already available, with many more likely to be possible in the not too distant future. Which traits should be selected and how they should be selected are topics of much debate within the worldwide medical community. The ethical and moral line is not always clear or agreed upon, and some fear that modern reproductive technologies could lead to a new form of eugenics.
Eugenics is the use of information and technology from a variety of sources to improve the genetic makeup of the human race. The goal of creating genetically superior humans was quite prevalent (although controversial) in several countries during the early 20th century, but fell into disrepute when Nazi Germany developed an extensive eugenics program in the 1930's and 40's. As part of their program, the Nazis forcibly sterilized hundreds of thousands of the so-called "unfit" and killed tens of thousands of institutionally disabled people as part of a systematic program to develop a genetically superior race of Germans known as Aryans. Ever since, eugenic ideas have not been as publicly expressed, but there are still those who promote them.
Efforts have been made in the past to control traits in human children using donated sperm from men with desired traits. In fact, eugenicist Robert Klark Graham established a sperm bank in 1980 that included samples exclusively from donors with high IQs. The "genius" sperm bank failed to capture the public's imagination and the operation closed in 1999.
In more recent times, the procedure known as prenatal genetic diagnosis (PGD) has been developed. PGD involves the screening of human embryos as part of the process of in vitro fertilization, during which embryos are conceived and grown outside the mother's body for some period of time before they are implanted. The term PGD usually refers to both the diagnosis, selection, and the implantation of the selected embryos.
In the least controversial use of PGD, embryos are tested for the presence of alleles which cause genetic diseases such as sickle cell disease, muscular dystrophy, and hemophilia, in which a single disease-causing allele or pair of alleles has been identified. By excluding embryos containing these alleles from implantation into the mother, the disease is prevented, and the unused embryos are either donated to science or discarded. There are relatively few in the worldwide medical community that question the ethics of this type of procedure, which allows individuals scared to have children because of the alleles they carry to do so successfully. The major limitation to this procedure is its expense. Not usually covered by medical insurance and thus out of reach financially for most couples, only a very small percentage of all live births use such complicated methodologies. Yet, even in cases like these where the ethical issues may seem to be clear-cut, not everyone agrees with the morality of these types of procedures. For example, to those who take the position that human life begins at conception, the discarding of unused embryos, a necessary result of PGD, is unacceptable under any circumstances.
A murkier ethical situation is found in the selection of a child's sex, which is easily performed by PGD. Currently, countries such as Great Britain have banned the selection of a child's sex for reasons other than preventing sex-linked diseases. Other countries allow the procedure for "family balancing", based on the desire of some parents to have at least one child of each sex. Still others, including the United States, have taken a scattershot approach to regulating these practices, essentially leaving it to the individual practicing physician to decide which practices are acceptable and which are not.
Even murkier are rare instances of disabled parents, such as those with deafness or dwarfism, who select embryos via PGD to ensure that they share their disability. These parents usually cite many positive aspects of their disabilities and associated culture as reasons for their choice, which they see as their moral right. To others, to purposely cause a disability in a child violates the basic medical principle of Primum non nocere, "first, do no harm." This procedure, although not illegal in most countries, demonstrates the complexity of ethical issues associated with choosing genetic traits in offspring.
Where could this process lead? Will this technology become more affordable and how should it be used? With the ability of technology to progress rapidly and unpredictably, a lack of definitive guidelines for the use of reproductive technologies before they arise might make it difficult for legislators to keep pace once they are in fact realized, assuming the process needs any government regulation at all. Other bioethicists argue that we should only deal with technologies that exist now, and not in some uncertain future. They argue that these types of procedures will always be expensive and rare, so the fears of eugenics and "master" races are unfounded and overstated. The debate continues.
Summary
The early stages of embryonic development begin with fertilization. The process of fertilization is tightly controlled to ensure that only one sperm fuses with one egg. After fertilization, the zygote undergoes cleavage to form the blastula. The blastula, which in some species is a hollow ball of cells, undergoes a process called gastrulation, in which the three germ layers form. The ectoderm gives rise to the nervous system and the epidermal skin cells, the mesoderm gives rise to the muscle cells and connective tissue in the body, and the endoderm gives rise to columnar cells and internal organs.
Glossary
- acrosomal reaction
- series of biochemical reactions that the sperm uses to break through the zona pellucida
- blastocyst
- structure formed when cells in the mammalian blastula separate into an inner and outer layer
- gastrulation
- process in which the blastula folds over itself to form the three germ layers
- holoblastic
- complete cleavage; takes place in cells with a small amount of yolk
- inner cell mass
- inner layer of cells in the blastocyst
- meroblastic
- partial cleavage; takes place in cells with a large amount of yolk
- polyspermy
- condition in which one egg is fertilized by multiple sperm
- trophoblast
- outer layer of cells in the blastocyst
- zona pellucida
- protective layer of glycoproteins on the mammalian egg